​​
​
​
Enhancing Claus SRU Efficiency with Feedforward Air Control
​
In sulfur recovery operations, particularly those employing the Claus process, controlling combustion air precisely is essential for maintaining high recovery rates and minimizing emissions. A feedforward control scheme—leveraging real-time analysis of acid gas upstream of the reaction furnace—provides a faster and more reliable means of adjusting air demand, especially during transitions or disturbances in process conditions.
When paired with modern, high-speed process analyzers and advanced control systems, this strategy significantly improves responsiveness and stabilizes furnace operation under both steady and non-steady-state conditions.
​
Fundamentals of the Claus Process and Air Control
The Claus process is based on the partial combustion of hydrogen sulfide (Hâ‚‚S) to form sulfur dioxide (SOâ‚‚), followed by catalytic conversion of Hâ‚‚S and SOâ‚‚ into elemental sulfur. Achieving and maintaining the ideal molar ratio of 2:1 (Hâ‚‚S:SOâ‚‚) is critical for optimal sulfur recovery.
Many factors can influence SRU efficiency—catalyst condition, process temperature, and flow rates, for example—but air control is the most dynamic. Even slight imbalances in combustion air can lead to unconverted Hâ‚‚S or SOâ‚‚ in the tail gas, reducing sulfur yield and increasing emissions.
​
Two-Tier Air Control: Feedforward and Feedback Loops
Primary Control: Feedforward Flow Ratio
The primary means of controlling combustion air involves a feedforward strategy: air flow is adjusted based on the acid gas flow rate, assuming a fixed composition. This method typically accounts for 90% or more of the required combustion air.
However, this strategy assumes the composition of acid gas streams remains stable—a significant limitation when feed composition changes rapidly or comes from multiple variable sources.
​
Secondary Control: Feedback Trim
To fine-tune the system, a feedback loop using tail gas analysis is employed. It measures the actual [Hâ‚‚S]/[SOâ‚‚] ratio and adjusts a trim air valve to restore balance when deviations occur. This method is highly accurate, but its effectiveness is limited by an inherent process lag time of 30 seconds or more. During that delay, any major change in feed composition can lead to inefficient combustion and upset conditions in the reaction furnace.
​​
​​
​
​
​
Why Feedback Alone Isn’t Enough
Because the feedback loop reacts only after a deviation is detected, it's often too slow to correct for fast composition shifts. In such cases, air control systems can fall out of sync with the process, leading to significant excursions from the target ratio, lower sulfur recovery, and higher pollutant levels in the tail gas.
​
​
​
AAI's Heated Acid Gas (HAG) Probe for single point for inlet and return
Role of Real-Time Feed Gas Analysis
To overcome the limitations of both static feedforward and delayed feedback control, real-time, multi-component gas analysis at the feed point is essential. By accurately measuring key combustion-driving components in the acid gas within seconds, the system can proactively adjust air input before imbalances occur.
​
​
​
​ AAI Heated Acid Gas Flow Schematics
​
Key components to monitor include:
​
Hydrogen Sulfide (Hâ‚‚S)
As the primary fuel in the Claus reaction, Hâ‚‚S concentration directly determines the combustion air requirement. Accurate, rapid Hâ‚‚S measurement allows for precise, real-time air demand estimation.
Total Hydrocarbons (THC)
Though typically present in small concentrations, hydrocarbons are fast-burning and demand significant oxygen. Rather than attempting to speciate every hydrocarbon—which can take minutes—measuring THC as a whole allows for a fast, practical estimation of their collective combustion impact.
Ammonia (NH₃)
Ammonia may be present in sour water stripper gas. It contributes to total air demand and can produce nitrogen oxides (NOx) if combustion is not properly managed. Including NH₃ in feed gas analysis ensures a more accurate air requirement calculation.
Carbon Dioxide (COâ‚‚)
COâ‚‚ does not directly affect oxygen demand but plays an indirect role. It can influence the formation of COS (carbonyl sulfide), especially if hydrolysis in the first converter bed is limited. Additionally, it alters the physical properties of the acid gas—such as molecular weight and specific gravity—affecting flow metering and air-fuel ratio calculations.
Water Vapor (Hâ‚‚O)
While moisture does not consume oxygen, it can influence gas flow characteristics and impact analyzer performance. Accurate measurement helps adjust for these effects, improving the reliability of gas flow and composition data.
​
Benefits of Real-Time Analysis and Feedforward Control Integration
By integrating fast gas analysis into a feedforward control loop, SRUs gain significant advantages:
-
Improved Process Stability: The control system can adapt to sudden feed changes instantly, maintaining an optimal air-to-fuel ratio.
-
Higher Sulfur Recovery Efficiency: Keeping the 2:1 Hâ‚‚S to SOâ‚‚ ratio stable minimizes loss of valuable sulfur.
-
Reduced Emissions: Better air control reduces excursions that cause increased SOâ‚‚ or Hâ‚‚S emissions in tail gas.
-
Minimized Process Upsets: Real-time data allows proactive rather than reactive control, reducing furnace instability and potential equipment stress.​
Conclusion
​
Modern feedforward control strategies, empowered by real-time feed gas analysis, represent a critical advancement in sulfur recovery unit performance. By responding to process changes before they affect downstream reactions, operators can achieve greater efficiency, reduced environmental impact, and more reliable operation.
​
​

Check out the AAI TLG Air Demand Analyzer in Action!
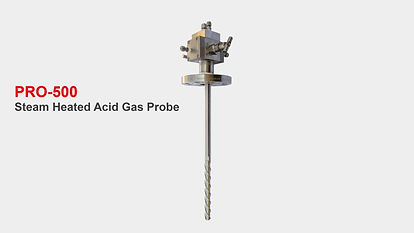
